Femtocells have been around since 2007. Before Femtocells, the smallest possible cell was the picocell that was designed to serve a small area, generally a office or a conference room. With Femtocells came the idea of having really small cells that can be used in houses and they were designed to serve just one home. Ofcourse in my past blogs you would have noticed me mentioning about Super Femtos and Femto++ that can cater for more users in a small confined space, typically a small office or a meeting room but as far as the most common definition is concerned they are designed for small confined spaces and are intended to serve less than 10 users simultaneously.
This blog post is based on IEEE paper on "Standardization of Femtocells in 3GPP" that appeared in IEEE Communications Magazine, September 2009 issue. This is not a copy paste article but is based on my understanding of Femtos and the research based on the IEEE paper. This post only focusses on 3GPP based femtocells, i.e., Femtocells that use UMTS HSDPA/HSPA based technology and an introduction to OFDM based LTE femtocells.
The reason attention is being paid to the Femtocells is because as I have blogged in the past, there are some interesting studies that suggest that majority of the calls and data browsing on mobiles originate in the home and the higher the frequency being used, the less its ability to penetrate walls. As a result to take advantage of the latest high speed technologies like HSDPA/HSUPA, it makes sense to have a small cell sitting in the home giving ability to the mobiles to have high speed error free transmission. In addition to this if some of the users that are experiencing poor signal quality are handed over to these femtocells, the overall data rate of the macro cell will increase thereby providing better experience to other users.
Each technology brings its own set of problems and femocells are no exception. There are three important problems that needs to be answered. They are as follows:
• Radio interference mitigation and management: Since femtocells would be deployed in adhoc manner by the users and for the cost to be kept down they should require no additional work from the operators point of view, they can create interference with other femtocells and in the worst possible scenario, with the macro cell. It may not be possible initially to configure everything correctly but once operational, it should be possible to adjust the parameters like power, scrambling codes, UARFCN dynamically to minimise the interference.
• Regulatory aspects: Since the mobiles work in licensed spectrum bands, it is required that they follow the regulatory laws and operate in a partcular area in a band it is licensed. This is not a problem in Europe where the operators are given bands for the whole country but in places like USA and India where there are physical boundaries within the country for the allocation of spectrum for a particular operator. This brings us to the next important point.
• Location detection: This is important from the regulatory aspect to verify that a Femtocell can use a particular band over an area and also useful for emergency case where location information is essential. It is important to make sure that the user does not move the device after initial setup and hence the detection should be made everytime the femto is started and also at regular intervals.
3GPP FEMTOCELLS STANDARDIZATION
Since the femtocells have been available for quite a while now, most of them do not comply to standards and they are proprietary solutions. This means that they are not interoperable and can only work with one particular operator. To combat this and to create economy of scale, it became necessary to standardise femtocells. Standardized interfaces from the core network to femtocell devices can potentially allow system operators to deploy femtocell devices from multiple vendors in a mix-and-match manner. Such interfaces can also allow femtocell devices to connect to gateways made by multiple vendors in the system operator’s core network (e.g., home NodeB gateway [HNB-GW] devices).
In 2008, Femto Forum was formed and it started discussion on the architecture. From 15 different proposals, consensus was reached in May over the Iuh interface as shown below.
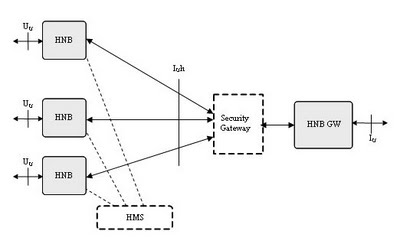
There are two main standard development organizations (SDOs) shaping the standard for UMTS-related (UTRAN) femto technology: 3GPP and The Broadband Forum (BBF).
More about 3GPP here. BBF (http://www.broadbandforum.org) was called the DSL Forum until last year. As an SDO to meet the needs of fixed broadband technologies, it has created specifications mainly for DSL-related technologies. It consists of multiple Working Groups. The Broadband Home WG in particular is responsible for the specification of CPE device remote management. The specification is called CPE wide area network (WAN) Management Protocol (CWMP), which is commonly known by its document number, TR-069.
There are several other important organisations for femto technology. The two popular ones are the Femto Forum (www.femtoforum.org) and Next Generation Mobile Network (NGMN).
3GPP has different terminology for Femtocells and components related to that. They are as follows:
Generic term: Femtocell
3GPP Term: home NodeB (HNB)
Definition: The consumer premises equipment (CPE) device that functions as the small-scale nodeB by interfacing to the handset over the standard air interface (Uu) and connecting to the mobile network over the Iuh interface.
Generic term: FAP Gateway (FAP-GW) or Concentrator
3GPP Term: home NodeB gateway (HNB-GW)
Definition: The network element that directly terminates the Iuh interface with the HNB and the existing IuCS and IuPS interface with the CN. It effectively aggregates a large number of HNBs (i.e., Iuh interface) and presents it as a single IuCS/PS interface to the CN.
Generic term: Auto-Configuration Server (ACS)
3GPP Term: home NodeB management system (HMS)
Definition: The network element that terminates TR-069 with the HNB to handle the remote management of a large number of HNBs.
In addition, there is a security gateway (SeGW) that establishes IPsec tunnel to HNB. This ensures that all the Iuh traffic is securely protected from the devices in home to the HNB-GW.
The HNB-GW acts as a concentrator to aggregate a large number of HNBs which are logically represented as a single IuCS/IuPS interface to the CN. In other words, from the CN’s perspective, it appears as if it is connected to a single large radio network controller (RNC). This satisfies a key requirement from 3GPP system operators and many vendors that the femtocell system architecture not require any changes to existing CN systems.
The radio interface between HNB and UE is the standard RRC based air interface but has been modified to incude HNB specific changes like the closed subscriber group (CSG) related information.
Two new protocols were defined to address HNB-specific differences from the existing Iu interface protocol to 3GPP UMTS base stations (chiefly, RANAP at the application layer).
• HNB Application Protocol (HNBAP): An application layer protocol that provides HNB-specific control features unique to HNB/femtocell deployment (e.g., registration of the HNB device with the HNBGW).
• RANAP User Adaptation (RUA): Provides a lightweight adaptation function to allow RANAP messages and signaling information to be transported directly over Stream Control Transport Protocol (SCTP) rather than Iu, which uses a heavier and more complex protocol stack that is less well suited to femtocells operating over untrusted networks from home users (e.g., transported over DSL or cable modem connections).
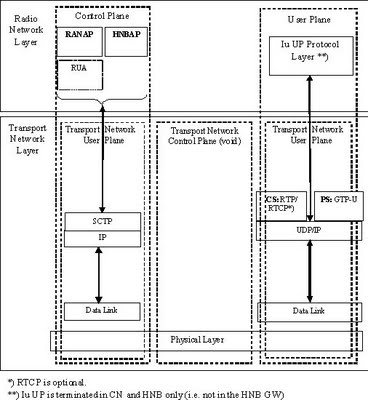
Figure above is representation of the protocol stack diagram being used in TS 25.467.
Security for femtocell networks consists of two major parts: femtocell (HNB) device authentication, and encryption/ciphering of bearer and control information across the untrusted Internet connection between the HNB and the HNB-GW (e.g., non-secure commercial Internet service). The 3GPP UMTS femtocell architecture provides solutions to both of these problems. 3GPP was not able to complete the standardization of security aspects in UMTS Release 8; however, the basic aspects of the architecture were agreed on, and were partially driven by broad industry support for a consensus security architecture facilitated in discussions within the Femto Forum. All security specifications will be completed in UMTS Release 9 (targeted for Dec. 2009).
FEMTOCELL MANAGEMENT
Management of femtocells is a very big topic and very important one for the reasons discussed above.
The BBF has created CWMP, also referred to as TR-069. TR-069 defines a generic framework to establish connection between the CPE and the automatic configuration server (ACS) to provide configuration of the CPE. The messages are defined in Simple Object Access Protocol (SOAP) methods based on XML encoding, transported over HTTP/TCP. It is flexible and extensive enough to incorporate various types of CPE devices using various technologies. In fact, although TR-069 was originally created to manage the DSL gateway device, it has been adopted by many other types of devices and technologies.
The fundamental functionalities TR-069 provides are as follows:
• Auto-configuration of the CPE and dynamic service provisioning
• Software/firmware management and upgrade
• Status and performance monitoring
• Diagnostics
The auto-configuration parameters are defined in a data model. Multiple data model specifications exist in the BBF in order to meet the needs of various CPE device types. In fact, the
TR-069 data model is a family of documents that has grown over the years in order to meet the needs of supporting new types of CPE devices that emerge in the market. In this respect, femtocell is no exception. However, the two most common and generic data models are:
•
TR-098: “Internet Gateway Device Data Model for TR-069”
•
TR-106: “Data Model Template for TR-069-Enabled Devices”
HAND-IN AND FEMTO-TO-FEMTO HANDOVERS
The 3GPP specifications focused on handovers in only one direction initially — from femtocell devices to the macrocellular system (sometimes called handout). A conscious decision was made to exclude handover from the macrocellular system to the femtocell devices (sometimes called macro to femtocell hand-in). This decision was driven by two factors:
• There are a number of technical challenges in supporting hand-in with unmodified mobile devices and core network components.
• The system operator requirements clearly indicate that supporting handout is much more important to end users.
Nonetheless, there is still a strong desire to develop open, interoperable ways to support handin in an efficient and reliable manner, and the second phase of standards in 3GPP is anticipated to support such a capability.
NEXT-G EFFORTS
3GPP Release 8 defines the over-the-air radio signaling that is necessary to support LTE femtocells. However, there are a number of RAN transport and core network architecture, interface, and security aspects that will be addressed as part off 3GPP’s Release 9 work efforts. While it is preliminary as of the publication of this article, it seems highly likely that all necessary RAN transport and core network work efforts for
LTE femtocells will be completed in 3GPP
Release 9 (targeted for completion by the end of 2009).
3GPP STANDARDS ON FEMTOCELLS
[1] 3GPP TS 25.331: RRC
[2] 3GPP TS 25.367: Mobility Procedures for Home NodeB (HNB); Overall Description; Sage 2
[3] 3GPP TS 25.467: UTRAN Architecture for 3G Home NodeB; Stage 2
[4] 3GPP TS 25.469: UTRAN Iuh Interface Home NodeB (HNB) Application Part (HNBAP) Signaling
[5] 3GPP TS 25.468: UTRAN Iuh Interface RANAP User Adaption (RUA) Signaling
[7] 3GPP TS 25.104: Base Station (BS) Radio Transmission and Reception (FDD)
[8] 3GPP TS 25.141: Base Station (BS) Conformance Testing (FDD)
[9] 3GPP TR 25.967: FDD Home NodeB RF Requirements
[10] 3GPP TS 22.011: Service Accessibility
[11] 3GPP TS 22.220: Service Requirements for Home NodeB (HNB) and Home eNodeB (HeNB)
[12] 3GPP TR 23.830: Architecture Aspects of Home NodeB and Home eNodeB
[13] 3GPP TR 23.832: IMS Aspects of Architecture for Home NodeB; Stage 2
[14] 3GPP TS 36.300: E-UTRA and E-UTRAN; Overall Description; Stage 2
[15] 3GPP TR 33.820: Security of H(e)NB 3GPP TR 32.821: Telecommunication Management; Study of Self-Organizing Networks (SON) Related OAM Interfaces for Home NodeB
[16] 3GPP TS 32.581: Telecommunications Management; Home Node B (HNB) Operations, Administration, Maintenance and Provisioning (OAM&P); Concepts and Requirements for Type 1 Interface HNB to HNB Management System (HMS)
[17] 3GPP TS 32.582: Telecommunications Management; Home NodeB (HNB) Operations, Administration, Maintenance and Provisioning (OAM&P); Information Model for Type 1 Interface HNB to HNB Management System (HMS)
[18] 3GPP TS 32.583: Telecommunications Management; Home NodeB (HNB) Operations, Administration, Maintenance and Provisioning (OAM&P); Procedure Flows for Type 1 Interface HNB to HNB Management System (HMS)
[19] 3GPP TS 32.584: Telecommunications Management; Home NodeB (HNB) Operations, Administration, Maintenance and Provisioning (OAM&P); XML Definitions for Type 1 Interface HNB to HNB Management System (HMS)
I would strongly recommend reading [3] and [6] for anyone who wants to gain better understanding of how Femtocells work.